The amazing layer of film! How does PVB laminated glass "work"?
Release time:
2021-01-28
In recent years, due to the rapid development of nanodispersion technology and magnetron sputtering technology, the production process of PVB interlayer glass has been enhanced through the addition, processing, and treatment of the interlayer, giving it spectral selectivity. This is particularly effective in absorbing or reflecting near-infrared light in the solar spectrum, significantly reducing the near-infrared transmittance ratio, and thus lowering the total solar transmittance SHGC.
In recent years, due to the rapid development of nano-dispersion technology and magnetron sputtering technology, the production process of the PVB interlayer in laminated glass has been enhanced by adding, processing, and treating materials, resulting in the interlayer having spectral selectivity, especially with good absorption or reflection performance for near-infrared light, significantly reducing the near-infrared transmittance ratio, thereby lowering the solar total transmittance ratio SHGC.
Currently, there are generally three methods for forming PVB combinations:
The first method involves adding heat-absorbing materials, such as nano-sized metal oxides, to the plasticizer of polyvinyl butyral (PVB). By adjusting the size and proportion of the added nano-particles, the interlayer can absorb near-infrared thermal energy.
The second method involves sandwiching a thermal insulation film between two layers of PVB glue. The thermal insulation film is a metal reflective film made using magnetron sputtering technology, which has high reflectivity for near-infrared light. The advantage is that it can reflect most of the infrared thermal energy, reducing the heat transfer from the film layer to the interior after heat absorption, thus lowering the solar total transmittance ratio SHGC. The main disadvantage of this PVB interlayer is its high cost, and its mechanical properties in laminated glass are also questioned in the industry.
The third method uses a shading type Low-E glass to replace one piece of clear glass, bonding it with ordinary PVB film through hot pressing, utilizing the reflective characteristics of the shading type Low-E film to block near-infrared light.
Since the near-infrared spectrum is adjacent to the visible light region, to ensure a high visible light transmittance, the stopband filter slope at the spectral boundary wavelength of 780nm needs to be as steep as possible (close to 90 degrees). This type of PVB interlayer requires offline sputtering of double silver or triple silver multilayer reflective films. Because these films are easily eroded and oxidized by moisture, they must be used in a dry gas environment, such as in hollow or vacuum glass. When used alone as laminated glass, the Low-E film layer must be bonded to the interlayer. However, this combination brings three problems: first, after bonding with the interlayer, the thermal/exchange coefficient is much greater than the radiative heat exchange of the Low-E film layer, effectively short-circuiting the Low-E film layer and losing its contribution to the heat transfer coefficient U value. Second, the mechanical properties (tensile strength, shear strength, etc.) of the multi-silver Low-E film layer are poor, reducing the strength of the laminated glass. Third, moisture intrusion makes it easier for the Low-E film layer to oxidize, further reducing the mechanical and optical performance of the laminated glass.
For the first scheme, incorporating nano-sized composite metal oxides into the PVB mainly focuses on the absorption of near-infrared thermal energy, part of which is transmitted to the interior through the inner glass. Therefore, each of the three schemes has its advantages and disadvantages. This article aims to provide references for laminated glass manufacturers when selecting interlayer schemes through photothermal calculations of different technical solutions.
1. Solar Radiation Energy
Understanding the spectral energy distribution of solar radiation is crucial for achieving optical energy savings through spectral filtering. For example, the irradiance of the red spectrum exceeds that of the blue spectrum by more than twice, clearly indicating that blocking the red spectrum contributes much more to reducing the total radiation entering the interior than blocking the blue spectrum.
Solar radiation energy is mainly distributed in the visible light region with wavelengths of 0.38um to 0.78um, and in the infrared region from 0.78um to 4um, with the former accounting for about 50% and the latter about 44%. The solar radiation in the ultraviolet region is minimal, only accounting for 6% of the total. More than 99% of the total radiation energy is within the wavelength range of 0.15 to 4μm.
After passing through the atmosphere, the intensity and spectral energy distribution of solar radiation change. The solar radiation energy that reaches the ground is much smaller than that at the upper boundary of the atmosphere, with a very small energy distribution in the ultraviolet spectrum, about 4%, while in the visible spectrum it is 48%, and in the infrared spectrum it is also 48%.
Scientific calculations commonly use the solar angle of incidence deviating from the zenith by 46.8 degrees, represented as AM1.5G, to indicate the solar spectral energy at the ground, with an irradiance of 963.75 watts/m². Indoor heating is influenced by the natural environment, including direct solar radiation in the wavelength range of 0.295 to 2.5μm, as well as the portion of heat absorbed and re-radiated by objects around 10um.
Due to the varying solar radiation intensity in different regions, for the convenience of thermal calculations and product standard formulation, a unified solar radiation heat gain factor SHGF (Solar Heat Gain Factor) is generally used when calculating the sum of heat transfer introduced by indoor and outdoor temperature differences and solar radiation. Its meaning is the solar radiation energy passing through 3mm thick ordinary glass in a unit time at that time and place, measured in W/m², and expressed as the product of Sc and SHGF, representing the total heat transferred through a unit area of glass in a unit time and the heat absorbed by the glass that is re-radiated indoors. For example, under ASHRAE summer standard conditions, SHGF is taken as 630W/m²; the expression for relative heat gain is:
RHG=14°F x U(summer)+200 x Sc(BTU/h-ft²)=7.78℃ x U(summer)+630 x Sc(W/m²)
The following provides some calculations and results for the heat absorption temperature rise, energy-saving effects, shading ratios, etc., of various combinations of laminated glass, especially clarifying misconceptions and concerns regarding the excessive temperature rise of glass due to heat absorption from heat-absorbing interlayers for some laminated glass manufacturing enterprises and users. The set values used in the calculations are derived from the technical parameters of some typical products and do not target all products.
2. Double Clear Glass Combination Laminated Glass:
i) Clear Glass + Heat-Absorbing Interlayer + Clear Glass
· The product technical parameters are set as follows:
Visible light direct transmittance ratio: 0.78;
Near-infrared direct transmittance ratio: 0.08;
Direct solar reflection ratio (300nm to 2500nm): 0.08;
· Other parameters are set as follows:
Indoor heat transfer coefficient αi: 8.7W/m²K;
Outdoor heat transfer coefficient αe: 23 W/m²K;
Total solar radiation energy: 630 x 0.87 = 548W/m²;
In the total radiation energy, visible light, infrared + ultraviolet each account for 50%;
The direct transmission ratio of visible light is 0.78, accounting for 39% of the total solar radiation; the direct transmission ratio of near-infrared is 0.08, accounting for 4%; the direct reflection ratio of sunlight is 0.08, totaling: 51%, with the remaining 49% being absorbed by the interlayer and glass, amounting to 268.5W/m².
The solar radiation heat absorbed by the interlayer is transferred to both sides of the glass (as shown in Figure 4). Due to the small temperature difference on both sides of the glass relative to the glass's temperature rise from heat absorption, it is negligible when calculating the temperature rise of the glass. Let the surface temperature of the glass on both sides in a steady state be T, with the indoor side temperature difference as △Ti and the outdoor side temperature difference as △Te. Since the heat transfer coefficients differ with temperature difference, the heat flow density transferred from the interlayer to both sides also differs, with more heat being transferred to the outdoor side with a larger heat exchange than to the indoor side with a smaller heat exchange. In thermal steady state:
αi△Ti + αe△Te = 268.5W/m²;
△Ti = T - Ti; △Te = T - Te;
Where: Ti and Te are the indoor and outdoor temperatures, respectively; αi and αe are the indoor and outdoor heat transfer coefficients, respectively;
a. When Ti = Te; △Ti = △Te = △T
△T(8.7 + 23) = 268.5;
△T = 268.5 / 31.7 = 8.47℃;
b. Indoor environment temperature 24℃, outdoor environment temperature 32℃
αi△Ti + αe△Te = 268.5W/m²;
8.7(T - 24) + 23(T - 32) = 268.5;
T = 38.3℃;
When the environmental temperatures on both sides are equal, the heat absorbed by the glass and transferred to the indoor side in steady state is 74W/m², accounting for 13.5% of the total radiation energy:
Glass temperature rise: 8.5℃;
Near-infrared transmission ratio: 0.35;
SHGC: 0.56;
Sc(SHGC/0.87): 0.65;
Light-heat ratio: 1.38;
When the indoor temperature is lower than the outdoor temperature, such as indoor 24℃ and outdoor 32℃, the temperature difference between the glass and the indoor environment increases, and the heat absorbed by the glass and transferred to the indoor side increases to 124W/m², accounting for 22.4% of the total radiation energy.
Glass surface temperature: 38.3℃;
Near-infrared transmission ratio: 52.8%;
SHGC: 0.65;
Sc(SHGC/0.87): 0.75;
Light-heat ratio: 1.1.
In terms of the temperature of heat-absorbing laminated glass, when the indoor and outdoor environmental temperatures are equal, the glass surface temperature rise is 8.5℃. In the case of indoor 24℃ and outdoor 32℃, the glass surface temperature is 38.3℃. The double white glass combination of laminated glass does not contribute to reducing the U-value.
ii) White glass + reflective interlayer + white glass
· The product technical parameters are set as follows:
The direct transmission ratio of visible light: 0.60;
The direct transmission ratio of near-infrared: 0.10;
The direct reflection ratio of visible light: 0.20;
The direct reflection ratio of near-infrared: 0.75;
Other parameters are set according to the aforementioned values.
The reflective interlayer laminated glass has a visible light transmission ratio of 60%; near-infrared transmission ratio of 10%; near-infrared reflection ratio of 75%; visible light direct reflection ratio of 20%; totaling 82.5%. The remaining 17.5% is absorbed by the interlayer and glass, amounting to 96W/m². Indoor 24℃, outdoor 32℃:
8.7(T - 24) + 23(T - 32) = 96;
T = 1041 / 31.7 = 32.8℃
From the above calculations, the heat absorbed by the interlayer and transferred to the indoor side in steady state is 8.7(32.8 - 24) = 77W/m², accounting for 14% of the total radiation energy. The double white glass combination of reflective laminated glass does not contribute to reducing the U-value:
Glass temperature: 32.8℃;
Near-infrared transmission ratio: 0.38;
SHGC: 0.49;
Sc(SHGC/0.87): 0.56;
Light-heat ratio: 1.1
3. White glass + ordinary PVB + online Low-E white glass:
This combination uses ordinary white glass on one side and online Low-E glass on the other side of the laminated glass (Figure 5), with the online Low-E film facing outward and not bonded to the interlayer. Utilizing the low radiation of online Low-E, as well as the stable characteristics of the film layer being hard, firm, washable, not undergoing oxidation reactions, and suitable for direct exposure to indoor environments, further reduces the impact of solar radiation and the temperature difference between indoors and outdoors on indoor heating.
Image
Set the product technical parameters as follows:
The direct transmission ratio of visible light: 0.70;
The direct transmission ratio of near-infrared: 0.05;
Direct solar reflection ratio (300nm to 2500nm): 0.08;
The use of online Low-E glass aims to reduce the indoor heat transfer coefficient of the glass, thereby reducing the solar radiation entering indoors and the temperature difference between indoors and outdoors on indoor heating. The following calculations use the following simplified formula for the heat transfer coefficient:
αi = 6.12 × εi + 3.6
αe = 6.12 × εe + 17.9
εi - Indoor side glass emissivity: online Low-E takes 0.2;
εe - Outdoor side glass emissivity: ordinary white glass takes 0.84;
According to the above formula, it is calculated as follows:
Indoor glass heat/exchange coefficient αi=4.8;
Outdoor glass heat/exchange coefficient αe=23;
According to the above parameters, the total direct transmission and reflection of sunlight is 47%, with the remaining 53% being absorbed by the interlayer and glass, absorbing solar radiation of 299W/m².
αi△Ti+αe△Te=299W/m²;
4.8△Ti+23△Te=299W/m²
△Ti=T-Ti;
△Te=T-Te;
Where: Ti and Te are the indoor and outdoor temperatures, respectively; αi and αe are the indoor and outdoor heat transfer coefficients, respectively;
a. When Ti = Te; △Ti = △Te = △T
△T(4.8+23)=290;
△T=10.7℃;
b. Indoor environment temperature 24℃, outdoor environment temperature 32℃
αi△Ti+αe△Te=290W/m²;
4.8(T-24)+23(T-32)=290;
T=41.4℃;
When the environmental temperatures on both sides are equal, the temperature rise of the glass due to heat absorption by the interlayer is 10.7℃. The heat transferred indoors is 51.6W/m², accounting for 9% of the total radiation energy:
Glass temperature rise: 10.7℃
Near-infrared transmission ratio: 0.24;
SHGC: 0.47;
Sc(SHGC/0.87): 0.54;
Light-heat ratio: 1.5
U (summer): 3.3W/m²K
U (winter): 3.0 W/m²K
When the indoor temperature is lower than the outdoor temperature, the temperature difference on the indoor side increases, and the proportion of heat absorbed by the glass and transferred indoors increases. When the indoor temperature is 24℃ and the outdoor temperature is 32℃, the heat transferred inward is 83.4W, accounting for 15% of the total radiation energy.
Glass surface temperature: 41.4℃.
Near-infrared transmission ratio: 0.35;
SHGC: 0.53;
Sc(SHGC/0.87): 0.60
Light-heat ratio: 1.16.
U (summer): 3.3W/m²K
U (winter): 3.0 W/m²K
From the above calculation results and actual test results, using a combination of white glass and Low-E glass for heat-absorbing interlayers will reduce the SHGC value. This is because the interlayer absorbs near-infrared thermal energy and transfers it as long waves to both sides. When it reaches the Low-E film layer on the outer side of the glass, the low radiation characteristics of the film layer significantly reduce the heat exchange coefficient of the glass surface. For example, in this case, the heat exchange coefficient drops from 8.7W/m² to 4.8W/m², nearly halving, thus greatly reducing the heat absorbed and transferred to the indoor side. According to the law of energy conservation, a reduction in heat exchange on the indoor side must increase the heat exchange on the outdoor side, and the glass temperature will also increase, as in this case where the glass temperature rises by 2℃.
4. White glass + heat-absorbing interlayer + combination of white glass and Low-E hollow glass:
Considering the double white glass laminated glass as an outer glass, combined with Low-E hollow glass (Figure 6), it can also be viewed as the glass on one side of the Low-E hollow glass bonding with the outer glass through the interlayer. After the interlayer absorbs heat, it transfers it to the hollow glass through contact heat exchange, which is the same principle as the hollow glass first absorbing long waves and then reducing heat transfer, utilizing the low heat transfer characteristics of the hollow glass to minimize the heat absorbed by the interlayer.
Assuming the heat absorbed by the interlayer per unit area is Q, the glass temperature under steady-state balance at different indoor and outdoor temperatures can be calculated using the following formula:
Q=U△i+ αe△e = U(T-Ti)+ 23(T-Te)
The interlayer absorbs solar radiation thermal energy and converts it into long waves transmitted to both sides of the glass. The Low-E surface of the hollow glass has no difference in reducing indoor heat transfer on the second or third surface. Using hollow glass with a lower heat transfer coefficient is very important for insulation and heat resistance, so it is necessary to use high-transmittance offline Low-E glass with a lower emissivity, for example, using Low-E glass with an emissivity of 0.03, filling with inert gas can reduce the U value to below 1.3W/m²K, and when the visible light transmission ratio reaches 60%, the SHGC value can still be reduced to 0.4.
Heat-absorbing interlayers can achieve the highest visible light transmission ratio and the lowest near-infrared (direct) transmission ratio, while hollow glass can control the U value below 1W/m²K, which is no longer a challenge. This type of laminated hollow glass is also the best choice for safety glass, so it is believed that it will be widely promoted in the industry.
5. Conclusion and Outlook:
This article mainly discusses heat-insulating laminated glass, with the most important indicators being the visible light transmission ratio Tvis and the total solar transmission ratio SHGC, as well as the light-heat ratio Tvis/SHGC. The choice of solution mainly depends on whether it can meet the requirements for visible light transmission while selecting a combination that provides the highest light-heat ratio.
From theoretical, measured results, and practical use, nano-ceramic laminated glass can provide the highest visible light transmission ratio and light-heat ratio.
Heat-absorbing laminated glass will generate a temperature rise when absorbing solar radiation, but the extent of the temperature rise is not large. Calculations and actual tests show that even at an indoor temperature of 26℃ and an outdoor temperature of 40℃, in a normal atmospheric environment, the glass temperature will not exceed 45℃. Even when both indoor and outdoor temperatures are 40℃, the glass temperature will still be less than 48.5℃, which is far from the softening temperature of the interlayer and will not cause bursting.
From the above calculations, it can also be concluded that for every 1℃ increase in glass temperature, the heat dissipation on the inner and outer surfaces increases by about 30W/m². After deducting the glass transmission and reflection, the maximum heat absorbed by the interlayer is 60% of the maximum solar radiation. Even in some extreme environments where solar radiation reaches 600W/m², the glass temperature rise will not exceed 20℃.
When using a combination of white glass and Low-E, the heat-absorbing glass shows significant advantages, with SHGC lower than that of reflective laminated glass, and the increase in indoor-outdoor temperature difference has little effect on SHGC.
The reason why heat-absorbing laminated glass has a high visible light transmittance ratio and light-heat ratio is that its filtering characteristic curve is relatively steep, allowing it to retain more visible light. Moreover, it has a lower reflectance, which does not cause light pollution. In contrast, if the reflectance of reflective interlayers is too high in the visible light range, it can lead to light pollution and a decrease in light transmittance.
Conclusion: In an era with a strong demand for green energy saving and comfort, laminated glass that balances energy saving and comfort has a huge market prospect. According to the comparison results in Table 1, nano-ceramic interlayers can provide the highest visible light transmittance ratio, the lowest shading ratio, and the highest light-heat ratio, thus significantly enhancing the energy saving and comfort of laminated glass. When combined with Low-E insulated glass, it can also provide excellent thermal insulation performance.
Outlook: Nano-ceramic interlayers bring a significant improvement in energy saving and comfort to laminated glass. It is believed that this will not only drive the overall upgrade of automotive windshields and new energy vehicle glass but also have significant implications for laminated insulated glass that emphasizes greater safety and energy efficiency, promoting the advancement of the insulated glass industry.
References:
Chen Qigao, "Fundamentals of Building Thermal Physics". Xi'an Jiaotong University Press. 1991
Wang Ye, "Discussion on the Concept of Low-Emission Glass and Its Research and Application Issues" China Glass, 2007, Issue 1
Dong Zizhong, Xu Yongguang, Chen Qigao, Wen Yongling, Chongqing University Institute of Architectural Technology, "Simplified Calculation Method for Window Heat Transfer Coefficient" Insulation Materials and Building Energy Saving
Related News
Contact Information
Address: No.4 Wanhua Avenue, Ziling Town, Dongbao District, Jingmen City
Official website:http://www.msjpvb.com/
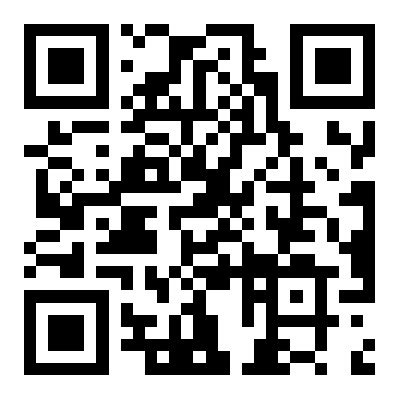
Mobile Access
Powered by www.300.cn This website supports IPV6 access